HP 234664-002 - ProLiant - ML330T02 Übersicht - Seite 5
Blättern Sie online oder laden Sie pdf Übersicht für Desktop HP 234664-002 - ProLiant - ML330T02 herunter. HP 234664-002 - ProLiant - ML330T02 20 Seiten. Visualization and acceleration in hp proliant servers
Auch für HP 234664-002 - ProLiant - ML330T02: Häufig gestellte Fragen (4 seiten), Handbuch zur Umsetzung (35 seiten), Technisches Weißbuch (12 seiten), Firmware-Aktualisierung (9 seiten), Handbuch zur Umsetzung (26 seiten), Einführung Handbuch (22 seiten), Handbuch zur Fehlersuche (18 seiten), Handbuch zur Umsetzung (11 seiten), Installationshandbuch (2 seiten), Konfigurationshandbuch (2 seiten), Einführung Handbuch (19 seiten), Handbuch aktualisieren (9 seiten), Handbuch aktualisieren (16 seiten), Einführung Handbuch (10 seiten), Installationsanleitung Handbuch (15 seiten), Technologie-Brief (9 seiten)
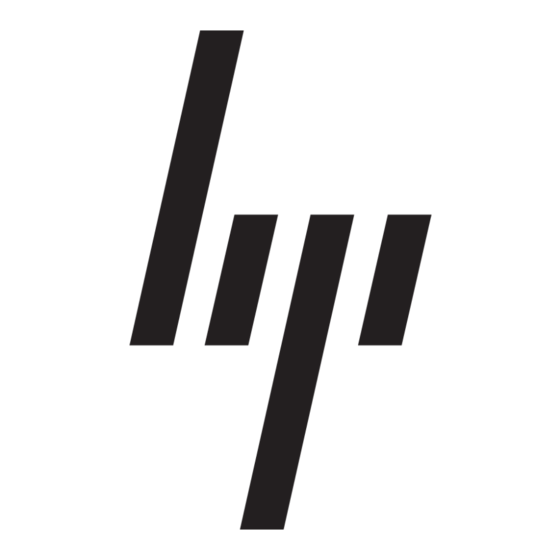